Ten years ago, Emmanuelle Charpentier and Jennifer Doudna published the study that paved the way for a new kind of genome editing: the suite of technologies now known as CRISPR. Writing in Science, they adapted an RNA-mediated bacterial immune defense into a targeted DNA-altering system. “Our study . . . highlights the potential to exploit the system for RNA-programmable genome editing,” they conclude in the abstract of their paper—a potential that, in the intervening years, transformed the life sciences.
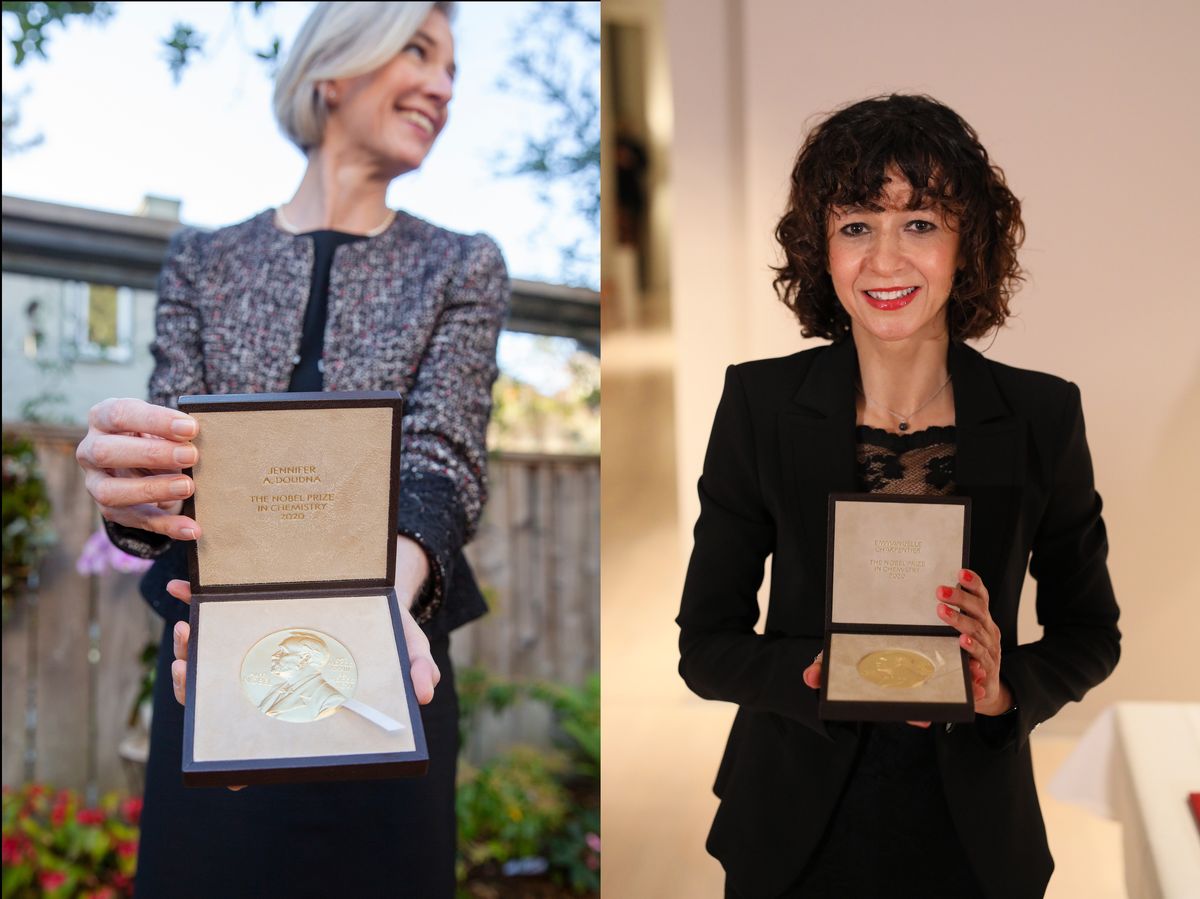
Jennifer Doudna (left) and Emmanuelle Charpentier (right) with their Nobel Prizes
© Nobel Prize Outreach. Left: Brittany Hosea-Small; Right: Bernhard Ludewig
From gene drives to screens, and diagnostics to therapeutics, CRISPR nucleic acids and the Cas enzymes with which they’re frequently paired have revolutionized how scientists tinker with DNA and RNA. Indeed, in 2020, Doudna and Charpentier were awarded the Nobel Prize in Chemistry for developing a technology that allows “rewriting the code of life,” as the secretary-general of the Royal Swedish Academy of Sciences described in his announcement. However, altering the code of life with CRISPR has been marred by ethical concerns. Perhaps the most prominent example was when Chinese scientist He Jiankui created the first gene edited babies using CRISPR/Cas9 genome editing. Doudna condemned Jiankui’s work, for which he was jailed, as “risky and medically unnecessary” and a “shocking reminder of the scientific and ethical challenges raised by this powerful technology.”
There’s also the fact that legal battles over who gets to claim ownership of the system’s many applications have persisted almost as long as the technology has been around. Both Doudna and Charpentier’s teams from the University of California, Berkeley, and the University of Vienna and a team led by the Broad Institute’s Feng Zhang claim to be the first to have adapted CRISPR-Cas9 for gene editing in complex cells (eukaryotes). Patent offices in different countries have reached varying decisions, but in the US, the latest rulings say that the Broad Institute of MIT and Harvard retains intellectual property of using CRISPR-Cas9 in eukaryotes, while Emmanuelle Charpentier, the University of California, and the University of Vienna maintain their original patent over using CRISPR-Cas9 for editing in vitro and in prokaryotes.
Still, despite the controversies, the technique continues to be explored academically and commercially for everything from gene therapy to crop improvement. Here’s a look at seven different ways scientists have utilized CRISPR.
Gene drives
Gene drives push offspring to inherit one allele more frequently than usual, enabling a trait to spread rapidly in a population. Such allele-spreading could allow scientists to genetically control invasive species or introduce resistance into disease vectors. But generating gene drives proved tricky. They were attempted before CRISPR came along, but generating the transgenic strains was an onerous process. CRISPR sped up their development, and thanks to the tech, researchers have finally created successful gene drives. For instance, in 2015, scientists created a large population of mosquitoes resistant to the malaria parasite. Also, the first successful gene drive in mammals was realized in 2019 when researchers engineered mice whose offspring were more likely than not to glow red under their white fur.
Genetic screens
Up until the advent of CRISPR, RNA interference (RNAi) was the primary technology used to identify mutations relevant to diseases or to assess potential drug targets in cells. Essentially, RNAi involves designing strands of RNA that bind to messenger RNAs and therefore block the production of protein from a given gene. But CRISPR allows for more efficient and specific targeting and inhibition, so CRISPR/Cas9 rapidly found use in large-scale genetic screens.
![]() SINGLE-CELL SCREEN: A library of guide RNAs—each targeting a unique gene for CRISPR-based interference and carrying a unique barcode sequence—is introduced into a population of cells at a concentration that results in one guide RNA entering one cell, on average. Individual cells are then sorted into droplets bearing uniquely barcoded polyT primers, which are used to extract the cell’s mRNA. Sequencing the RNA then reveals both the introduced genetic mutations—determined by the guide RNA—and the transcriptional effect of that perturbation—determined by the collection of mRNAs bearing the cell-specific barcode (from the polyT primer). © GEORGE RETSECK |
Combining CRISPR screens with organoids and barcoding technologies has, for example, led to the identification of new regulators of microcephaly, a condition in which babies are born with abnormally small heads, in 2021. Other researchers used CRISPR screens in 2016 to find genes that are necessary to develop effective immunotherapies for cancer, or to identify enhancers in noncoding DNA. Single-cell CRISPR screens, presented in 2016, even allow researchers to track responses to genetic changes in large numbers of individual cells.
Diagnostics, including COVID-19 tests
Because CRISPR can be designed to target specific genetic sequences, CRISPR-based platforms are being developed to quickly and cheaply detect pathogens including Zika, HPV, and Ebola. DETECTR, a diagnostic system developed in Jennifer Doudna’s lab and first presented in 2018, does so by detecting nucleic acid sequences. DETECTR uses a different Cas enzyme, Cas12a, which chops up single-stranded DNA after binding to its target sequence. Initially, the team targeted CRISPR/Cas12a to sequences of human papillomaviruses, including a reporter DNA molecule that glows when it is cut, making any positive samples easy to spot. A similar tool by MIT’s Feng Zhang, called SHERLOCK, has demonstrated the ability to distinguish between sequences that differ by a single point mutation, suggesting it could be used for genotyping DNA as well as identifying pathogen variants and cancerous mutations.
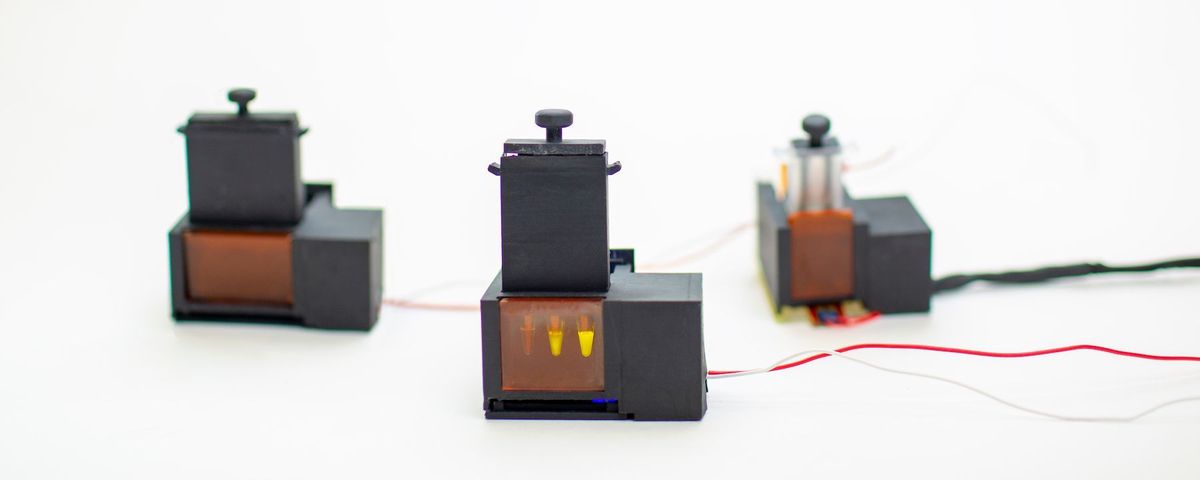
The miSHERLOCK device glows brightly if a saliva sample contains SARS-CoV-2 RNA.
WYSS INSTITUTE AT HARVARD UNIVERSITY
Of course, both SHERLOCK and DETECTR have been adapted to diagnose the infectious disease de jour: COVID-19. miSHERLOCK is a self-contained COVID-19 test, which runs the detection process from beginning to end within a 3D-printed device. Researchers have also combined DETECTR with LAMP, a method to amplify nucleic acid sequences without PCR. In positive tests, the Cas12 enzyme homes in on its target sequence and causes a chopping frenzy among nucleic acids. The enzyme also chops up a reporter molecule, which is detected on paper strips in positive tests. Another CRISPR-based test, initially developed as an assay for sickle cell anemia, was also repurposed into another test for COVID.
CRISPR’s target specificity can extend beyond nucleic acids. Yet another CRISPR-based system, called CAMERA, can record data from human and bacterial cells. In CAMERA, the guide molecule is produced only under certain conditions, such as the presence of antibiotics. Once it is produced, the CRISPR system snips certain DNA plasmids but not others. While the targeted plasmids are destroyed by the cell, the untargeted ones are not. By measuring the ratio between these two types of plasmids, the researchers can establish whether the cell was exposed to a certain condition. CRISPR tools, such as CAMERA, are also being investigated for biological sensors to detect compounds such as antibiotics, nutrients, or toxins.
Gene therapy
Gene editing also opens the door for gene therapy: the precise alteration of the genome to treat or prevent a condition. Gene therapy was first achieved in humans in 2017 using zinc finger nucleases, a gene editing technology that was popular prior to the development of CRISPR-Cas, which inserts genes more precisely and is therefore an appealing tool for such therapies. CRISPR was first used to edit a gene inside a living patient in 2020, when doctors in Portland injected a CRISPR-based therapy behind a patient’s retina to treat an inherited form of blindness. Other trials of CRISPR-based gene therapies are underway, including some to treat transthyretin amyloidosis and b-thalassemia, among other diseases.
Therapeutics
Therapeutic use of CRISPR editing doesn’t have to target a patient’s genome directly. Multiple efforts are underway to use CRISPR in other healing ways. For instance, the biotech company Eligo Bioscience is developing CRISPR-based methods to target Cutibacterium acnes bacteria carrying genes associated with acne vulgaris. Other researchers are using CRISPR to modify CAR T cells, a cancer immunotherapy in which a patient’s T cells are reprogrammed to mark cancer cells for destruction. However, these CRISPR-engineered CAR T cells, presented in 2020, haven’t yet reached the clinic.
Crop and livestock improvement
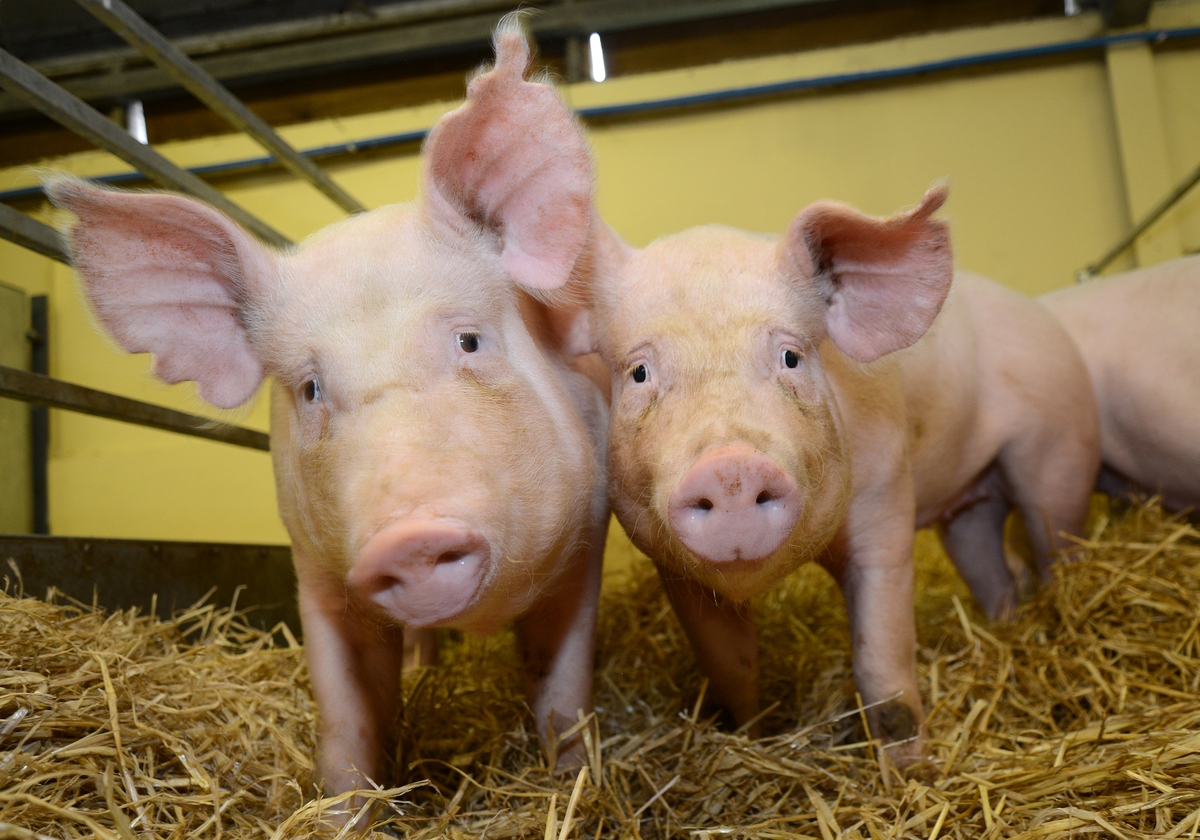
Pigs engineered using CRISPR to be resistant to PPRS virus
NORRIE RUSSELL
For as long as humans have farmed, there have been efforts to improve the plants and animals people raise for food. At first, these efforts relied on artificial selection and mutagenic processes to produce desirable, inheritable features. But now, scientists can generate organisms with higher yields, enhanced disease resistance, improved nutrient profiles, and other beneficial traits more precisely thanks to genome editing. And while technologies like zinc finger nucleases and TALENs (nucleases that introduce double-strand DNA breaks at target sites), can and have been used to genetically improve crops and livestock, these techniques are more expensive and less precise than CRISPR. Non-browning mushrooms, disease-resistant bananas and pigs, and corn with increased productivity are some of the CRISPR-based efforts underway by the agritech industry.
Basic research
CRISPR’s low cost, precision, and ease of use compared to other gene editing technologies has made it a go-to technique in a variety of research fields. CRISPR editing gave scientists a clue as to where giraffe’s strange body shape comes from, for example. In a 2021 study, they inserted the gene FGFRL1, the giraffes’ most divergent gene as compared to other ruminants, into mice, they found subtle changes indicating that giraffe’s FGFRL1 strengthens the bones and blood vessels to cope with their unique lifestyle. Researchers have also used CRISPR-edited Aedes aegypti mosquitoes to uncover specific compounds in human sebum that mark humans as attractive to the insects. And in a 2022 study, researchers reversed epigenetic markers using a sister system called CRISPR-d/Cas9 and decreased anxiety and alcohol-seeking in rats, suggesting that focusing on the epigenome could help treat people struggling with alcohol use disorder.
Post a Comment